First Ionisation Energy: The energy required to remove the most loosely held electron from one mole of gaseous atoms to produce 1 mole of gaseous ions each with a charge of 1+.
This is more easily seen in symbol terms.

It is the energy needed to carry out this change per mole of X.
Things to take note about the equation:The state symbols - (g) - are essential. When you are talking about ionisation energies, everything must be present in the gas state.
Ionisation energies are measured in kJ mol-1 (kilojoules per mole). They vary in size from 381 (which you would consider very low) up to 2370 (which is very high).
All elements have a first ionisation energy - even atoms which don't form positive ions in test tubes. The reason that helium (1st I.E. = 2370 kJ mol-1) doesn't normally form a positive ion is because of the huge amount of energy that would be needed to remove one of its electrons.
Patterns of first ionisation energies in the Periodic Table
The first 20 elements

First ionisation energy shows periodicity. That means that it varies in a repetitive way as you move through the Periodic Table. For example, look at the pattern from Li to Ne, and then compare it with the identical pattern from Na to Ar.
These variations in first ionisation energy can all be explained in terms of the structures of the atoms involved.
Factors affecting the size of ionisation energy:Ionisation energy is a measure of the energy needed to pull a particular electron away from the attraction of the nucleus. A high value of ionisation energy shows a high attraction between the electron and the nucleus.
The size of that attraction will be governed by:
The charge on the nucleus.The more protons there are in the nucleus, the more positively charged the nucleus is, and the more strongly electrons are attracted to it.
The distance of the electron from the nucleus.Attraction falls off very rapidly with distance. An electron close to the nucleus will be much more strongly attracted than one further away.
The number of electrons between the outer electrons and the nucleus.Consider a sodium atom, with the electronic structure 2,8,1. (There's no reason why you can't use this notation if it's useful!)
If the outer electron looks in towards the nucleus, it doesn't see the nucleus sharply. Between it and the nucleus there are the two layers of electrons in the first and second levels. The 11 protons in the sodium's nucleus have their effect cut down by the 10 inner electrons. The outer electron therefore only feels a net pull of approximately 1+ from the centre. This lessening of the pull of the nucleus by inner electrons is known as screening or shielding.
Whether the electron is on its own in an orbital or paired with another electron.Two electrons in the same orbital experience a bit of repulsion from each other. This offsets the attraction of the nucleus, so that paired electrons are removed rather more easily than you might expect.
Explaining the pattern in the first few elements
Hydrogen has an electronic structure of 1s1. It is a very small atom, and the single electron is close to the nucleus and therefore strongly attracted. There are no electrons screening it from the nucleus and so the ionisation energy is high (1310 kJ mol-1).
Helium has a structure 1s2. The electron is being removed from the same orbital as in hydrogen's case. It is close to the nucleus and unscreened. The value of the ionisation energy (2370 kJ mol-1) is much higher than hydrogen, because the nucleus now has 2 protons attracting the electrons instead of 1.
Lithium is 1s22s1. Its outer electron is in the second energy level, much more distant from the nucleus. You might argue that that would be offset by the additional proton in the nucleus, but the electron doesn't feel the full pull of the nucleus - it is screened by the 1s2 electrons.
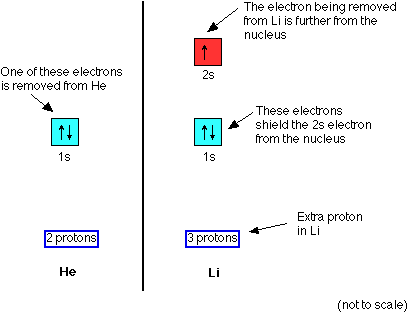
You can think of the electron as feeling a net 1+ pull from the centre (3 protons offset by the two 1s2 electrons).
If you compare lithium with hydrogen (instead of with helium), the hydrogen's electron also feels a 1+ pull from the nucleus, but the distance is much greater with lithium. Lithium's first ionisation energy drops to 519 kJ mol-1 whereas hydrogen's is 1310 kJ mol-1.
The patterns in periods 2 and 3Talking through the next 17 atoms one at a time would take ages. We can do it much more neatly by explaining the main trends in these periods, and then accounting for the exceptions to these trends.
The first thing to realise is that the patterns in the two periods are identical - the difference being that the ionisation energies in period 3 are all lower than those in period 2.
Explaining the general trend across periods 2 and 3The general trend is for ionisation energies to increase across a period.
In the whole of period 2, the outer electrons are in 2-level orbitals - 2s or 2p. These are all the same sort of distances from the nucleus, and are screened by the same 1s2 electrons.
The major difference is the increasing number of protons in the nucleus as you go from lithium to neon. That causes greater attraction between the nucleus and the electrons and so increases the ionisation energies. In fact the increasing nuclear charge also drags the outer electrons in closer to the nucleus. That increases ionisation energies still more as you go across the period.
In period 3, the trend is exactly the same. This time, all the electrons being removed are in the third level and are screened by the 1s22s22p6 electrons. They all have the same sort of environment, but there is an increasing nuclear charge.
Why the drop between groups 2 and 3 (Be-B and Mg-Al)?
The explanation lies with the structures of boron and aluminium. The outer electron is removed more easily from these atoms than the general trend in their period would suggest.
Be 1s22s2 1st I.E. = 900 kJ mol-1
B 1s22s22px1 1st I.E. = 799 kJ mol-1
You might expect the boron value to be more than the beryllium value because of the extra proton. Offsetting that is the fact that boron's outer electron is in a 2p orbital rather than a 2s. 2p orbitals have a slightly higher energy than the 2s orbital, and the electron is, on average, to be found further from the nucleus. This has two effects.
-The increased distance results in a reduced attraction and so a reduced ionisation energy.
-The 2p orbital is screened not only by the 1s2 electrons but, to some extent, by the 2s2 electrons as well. That also reduces the pull from the nucleus and so lowers the ionisation energy.
The explanation for the drop between magnesium and aluminium is the same, except that everything is happening at the 3-level rather than the 2-level.
Mg 1s22s22p63s2 1st I.E. = 736 kJ mol-1
Al 1s22s22p63s23px1 1st I.E. = 577 kJ mol-1
The 3p electron in aluminium is slightly more distant from the nucleus than the 3s, and partially screened by the 3s2 electrons as well as the inner electrons. Both of these factors offset the effect of the extra proton.
Why the drop between groups 5 and 6 (N-O and P-S)?Once again, you might expect the ionisation energy of the group 6 element to be higher than that of group 5 because of the extra proton. What is offsetting it this time?
N 1s22s22px12py12pz1 1st I.E. = 1400 kJ mol-1
O 1s22s22px22py12pz1 1st I.E. = 1310 kJ mol-1
The screening is identical (from the 1s2 and, to some extent, from the 2s2 electrons), and the electron is being removed from an identical orbital.
The difference is that in the oxygen case the electron being removed is one of the 2px2 pair. The repulsion between the two electrons in the same orbital means that the electron is easier to remove than it would otherwise be.
The drop in ionisation energy at sulphur is accounted for in the same way.
Trends in ionisation energy down a groupAs you go down a group in the Periodic Table ionisation energies generally fall. You have already seen evidence of this in the fact that the ionisation energies in period 3 are all less than those in period 2.
Taking Group 1 as a typical example:
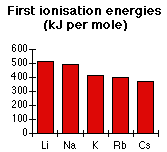
Why is the sodium value less than that of lithium?
There are 11 protons in a sodium atom but only 3 in a lithium atom, so the nuclear charge is much greater. You might have expected a much larger ionisation energy in sodium, but offsetting the nuclear charge is a greater distance from the nucleus and more screening.
Li 1s22s1 1st I.E. = 519 kJ mol-1
Na 1s22s22p63s1 1st I.E. = 494 kJ mol-1
Lithium's outer electron is in the second level, and only has the 1s2 electrons to screen it. The 2s1 electron feels the pull of 3 protons screened by 2 electrons - a net pull from the centre of 1+.
The sodium's outer electron is in the third level, and is screened from the 11 protons in the nucleus by a total of 10 inner electrons. The 3s1 electron also feels a net pull of 1+ from the centre of the atom. In other words, the effect of the extra protons is compensated for by the effect of the extra screening electrons. The only factor left is the extra distance between the outer electron and the nucleus in sodium's case. That lowers the ionisation energy.
Similar explanations hold as you go down the rest of this group - or, indeed, any other group.
Trends in ionisation energy in a transition series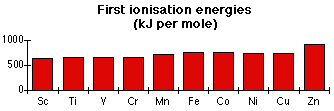
Apart from zinc at the end, the other ionisation energies are all much the same.
All of these elements have an electronic structure [Ar]3dn4s2 (or 4s1 in the cases of chromium and copper). The electron being lost always comes from the 4s orbital.
As you go from one atom to the next in the series, the number of protons in the nucleus increases, but so also does the number of 3d electrons. The 3d electrons have some screening effect, and the extra proton and the extra 3d electron more or less cancel each other out as far as attraction from the centre of the atom is concerned.
The rise at zinc is easy to explain.
Cu [Ar]3d104s1 1st I.E. = 745 kJ mol-1
Zn [Ar]3d104s2 1st I.E. = 908 kJ mol-1
In each case, the electron is coming from the same orbital, with identical screening, but the zinc has one extra proton in the nucleus and so the attraction is greater. There will be a degree of repulsion between the paired up electrons in the 4s orbital, but in this case it obviously isn't enough to outweigh the effect of the extra proton.
Ionisation energies and reactivityThe lower the ionisation energy, the more easily this change happens:

You can explain the increase in reactivity of the Group 1 metals (Li, Na, K, Rb, Cs) as you go down the group in terms of the fall in ionisation energy. Whatever these metals react with, they have to form positive ions in the process, and so the lower the ionisation energy, the more easily those ions will form.
The danger with this approach is that the formation of the positive ion is only one stage in a multi-step process.
For example, you wouldn't be starting with gaseous atoms; nor would you end up with gaseous positive ions - you would end up with ions in a solid or in solution. The energy changes in these processes also vary from element to element. Ideally you need to consider the whole picture and not just one small part of it.
However, the ionisation energies of the elements are going to be major contributing factors towards the activation energy of the reactions. Remember that activation energy is the minimum energy needed before a reaction will take place. The lower the activation energy, the faster the reaction will be - irrespective of what the overall energy changes in the reaction are.
The fall in ionisation energy as you go down a group will lead to lower activation energies and therefore faster reactions.